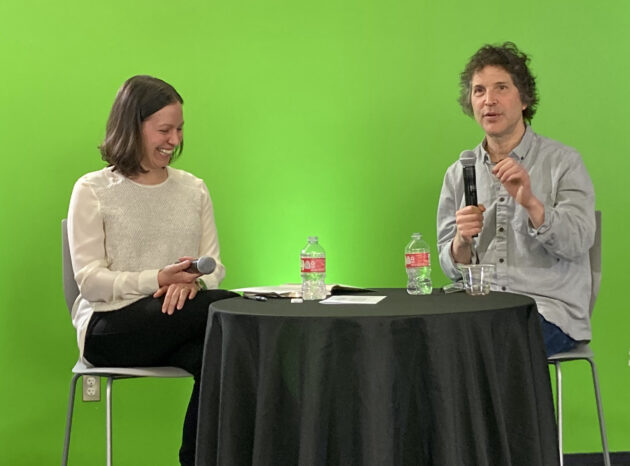
The Institute for Protein Design at the University of Washington is a startup factory, spinning out companies developing new protein-based therapeutics, industrial enzymes, materials, biosensors and more.
A key to the institute’s entrepreneurial success is a mindset of open inquiry and collaboration — and an emphasis on making the code for its protein-design tools readily available.
“I really believed from the beginning that we should share everything that we did,” said IPD director and UW professor David Baker, speaking at an event on campus in Seattle last week.
More than two decades ago, Baker and his colleagues developed an early protein design tool and made the code available through a consortium called Rosetta Commons. Commercial customers pay a licensing fee, while academics access the Rosetta code for free — and build and improve on it. More than 70 academic and industry organizations contribute to Rosetta Commons.
“That code really spread around the world. And it was basically what everybody used,” said Baker.
Other academic groups built similar protein design tools, but were more secretive because they wanted to start companies, said Baker. In the end, their software didn’t gain traction because it didn’t benefit from the high level of collaboration that Rosetta did, he said. The IPD’s openness led to better software, he said, and set the stage for later spinouts.
The IPD kept its focus on accessibility with the advent of artificial intelligence. A deep learning tool from Google’s DeepMind to predict how proteins fold hit the field in 2020, followed by a similar tool from the IPD. Both won Science magazine’s 2021 Breakthrough of the Year award.
Since then, the IPD has released new deep learning models that go further, and design new proteins from scratch. “They’re pretty much the industry standard,” said Baker of the new open-source tools.
“Tech companies are really good at what they do. But by being in a closed environment, it’s hard to innovate broadly,” he added. “In our space, making code freely available has been clearly the best thing I could imagine.”
The field overall would move even faster if more large, proprietary datasets at biopharma companies could be accessed to train deep learning models, Baker said.
Baker also credits the IPD for laboratory innovations that have enabled researchers to efficiently test their protein designs and feed the data back into their models to improve them.
The IPD has spun out nine companies, all based in Seattle. In 2020, Takeda bought IPD spinout PvP Biologics for $330 million and in February AstraZeneca completed its $1.1 billion acquisition of IPD spinout Icosavax, which develops vaccines.
Years ago, venture capitalists would insist that Baker start companies only in Boston. But the graduate of Seattle’s Garfield High School stayed loyal to his hometown, which he returned to after completing his Ph.D studies and postdoctoral work in the Bay Area.
“If you want to hire people who know how to do protein design, Seattle is definitely the best place,” said Baker. “I think you can kind of create an ecosystem just by sustained activity.”
Baker, who switched to science after first studying social studies in college, didn’t start out with the goal of becoming an entrepreneur. “You never know where life is going to take you,” said Baker, who advises his students to not plan too far ahead. “Being an entrepreneur and starting companies really grew out of the work that we were doing.”
The Q&A interview was hosted at the offices of UW’s commercialization arm CoMotion, and was conducted by Jenny Cronin, a principal at the AI2 Incubator in Seattle. Read more of Baker’s comments below. Questions and answers have been edited for brevity and clarity.
Jenny Cronin: How does your lab pull in new AI methods, such as diffusion models that are behind image generators like DALL-E? The IPD deep learning tool RFdiffusion is one example.
David Baker: We’re just incredibly open, so we have visitors coming through all the time. Whether they are a venture capitalist or someone from a pharma company, or a visiting student, they can see everything we’re doing all the time. We have a lot of people wanting to collaborate and visit, and they often bring in ideas. In the case of RFdiffusion, there was a very talented visiting student, and he started to play with diffusion models.
Do you have suggestions for how students can reach out and make those connections, to generate collaborations?
I think collaboration is just absolutely central to science. I encourage my students to just email people. If they’re working on a problem, I say figure out who the best three people are in the world and email them. Sometimes you won’t get an answer, but other times you will, and that can start a connection which could really transform your research.
What are some of the grand challenges in AI and protein design that we are poised to solve?
Antibodies are really the key molecules for protein therapeutics in the pharmaceutical industry. I think we’re going to see a transition from protein therapeutics being obtained by kind of a black magic — like immunizing an animal and letting the natural immune system come up with a solution — to actually being designed rationally. Rational methods like deep learning methods should in the long run be able to create antibodies that not only bind the target just as you want, but also ones that will be developable and safe and all that.
And then going beyond antibodies, what you’d really like are smarter therapeutics that can actually do calculations in the body and be responsive and only act in the right place, and not others. And I think that’s going to become increasingly possible as protein design methods become more powerful.
I’m curious to hear about non-therapeutic areas.
If you look in biology, proteins capture energy from the sun and use it to make molecules — photosynthesis is really a fascinating process. We’re working on, basically, artificial photosynthetic systems that will capture light and solar energy and use it to do things.
Another thing that proteins do very effectively is mineralization, such as in bones, teeth, and shells. That’s a very exciting area both for carbon fixation and making new types of materials. We’re also filling up the world with plastic, so we can develop catalysts that can break them down.
For diagnostics, one area we’re very excited about is being able to design nanopores that have completely controllable size and interiors. The applications of those are huge, such as for DNA or protein sequencing.
Do you do anything at the IPD to try to encourage entrepreneurial thinking?
The IPD has a translational investigator program where you stay on for a year or two after you finish your Ph.D., and develop what you’ve generated and make it better. People who have been successful in starting companies will also come back to the IPD. We have happy hours, and they give advice and so on. I also make sure that people are always talking to each other and I encourage people to form teams if they’re interested in starting a company — not having this image that you have got to just do it by yourself.
When you and students are choosing projects, how much do you balance commercial potential with interesting scientific research?
The key thing is there has to be a really important unsolved problem that should be solvable in the next couple of years. That’s a real art, though, to find those problems. And you can’t pick something that’s too easy — you have to really push the envelope. And if you choose a problem that’s way too hard then you won’t get anywhere either. But if you choose the right problems, where this is the time to solve them, a surprisingly large fraction will have some commercial potential — maybe in a way that you didn’t anticipate. And that certainly happened with a lot of the things we’ve done.